A long-period radio transient active for three decades
Congratulations to Manisha who was part of a recent paper published in Nature this month:
This object belongs to a new class of radio transients of which only 3 are now known. Manisha found one source and Natasha Hurley-Walker (ICRAR) found two sources.
Abstract
Several long-period radio transients have recently been discovered, with strongly polarized coherent radio pulses appearing on timescales between tens to thousands of seconds. In some cases, the radio pulses have been interpreted as coming from rotating neutron stars with extremely strong magnetic fields, known as magnetars; the origin of other, occasionally periodic and less-well-sampled radio transients is still debated. Coherent periodic radio emission is usually explained by rotating dipolar magnetic fields and pair-production mechanisms, but such models do not easily predict radio emission from such slowly rotating neutron stars and maintain it for extended times. On the other hand, highly magnetic isolated white dwarfs would be expected to have long spin periodicities, but periodic coherent radio emission has not yet been directly detected from these sources. Here we report observations of a long-period (21 min) radio transient, which we have labelled GPM J1839–10. The pulses vary in brightness by two orders of magnitude, last between 30 and 300 s and have quasiperiodic substructure. The observations prompted a search of radio archives and we found that the source has been repeating since at least 1988. The archival data enabled constraint of the period derivative to <3.6 × 10−13 s s−1, which is at the very limit of any classical theoretical model that predicts dipolar radio emission from an isolated neutron star.
Main
We monitored the galactic plane using the Murchison Widefield Array (MWA; see Methods) to detect ‘slow’ transients within 48 h of observation. From this search, we discovered the transient source GPM J1839–10, initially with two substantial detections of 30-s-wide pulses on a single night. As monitoring continued, we made several further detections and also started a continuous monitoring campaign with the MWA, as well as follow-up observations with the Australia Telescope Compact Array (ATCA), Parkes/Murriyang radio telescope, the Australian Square Kilometre Array Pathfinder (ASKAP) and MeerKAT.
Interferometric observations with MeerKAT constrained the (J2000) position to right ascension (RA) = 18 h 39 min 02.000 s, declination (dec.) = −10° 31′ 49.37″, with an uncertainty of 0.15″, whereas the high-time-resolution (3.9-ms) observing mode enabled a detailed view of the pulse structure and an accurate calculation of the dispersion measure (DM), 273.5 ± 2.5 pc cm−3. Using the YMW16 galactic free-electron density model, this corresponds to a distance of 5.7 ± 2.9 kpc for the line-of-sight towards GPM J1839–10. Over a typical pulse, the degree of linear polarization varies from 10% to 100%, with a generally flat phase angle. We observe bursts of duration 0.2–4 s in which this angle switches by 90°, resembling the orthogonal polarization modes (OPMs) commonly seen in neutron star pulsars (Fig. 1). Quasiperiodic oscillations are seen in many pulses, reminiscent of pulsar single-pulse microstructure, but two to three orders of magnitude larger in scale than expected (see Methods for more details on pulse polarization and substructure).
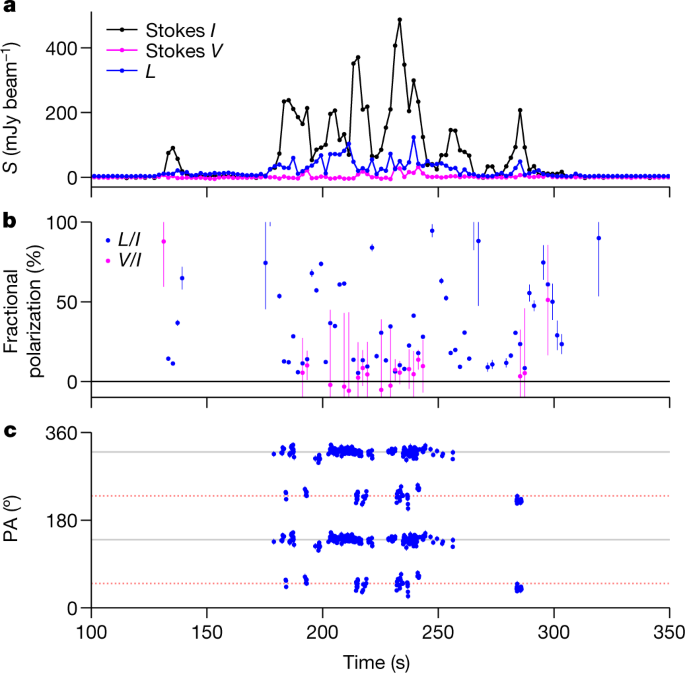
Searches of archival data yielded further detections as far back as 1988 by the Very Large Array (VLA), the VLA Low Band Ionospheric and Transient Experiment (VLITE) and the Giant Metrewave Radio Telescope (GMRT). Figure 2 shows the accumulated light curves from all observations, with maximum flux densities ranging from 0.1 to 10 Jy. The pulse widths range between 30 and 300 s and can appear anywhere within an approximately 400-s-wide window. The morphologies and brightnesses of the observed pulses change markedly with time, sometimes disappearing below detectability, even for the very sensitive MeerKAT observations. Depending on telescope sensitivity, this nulling fraction is 50–70%. No persistent radio source was detected down to a 3σ limit of 60 μJy beam−1 using MeerKAT ultra-high-frequency data integrated outside the pulse-activity windows.
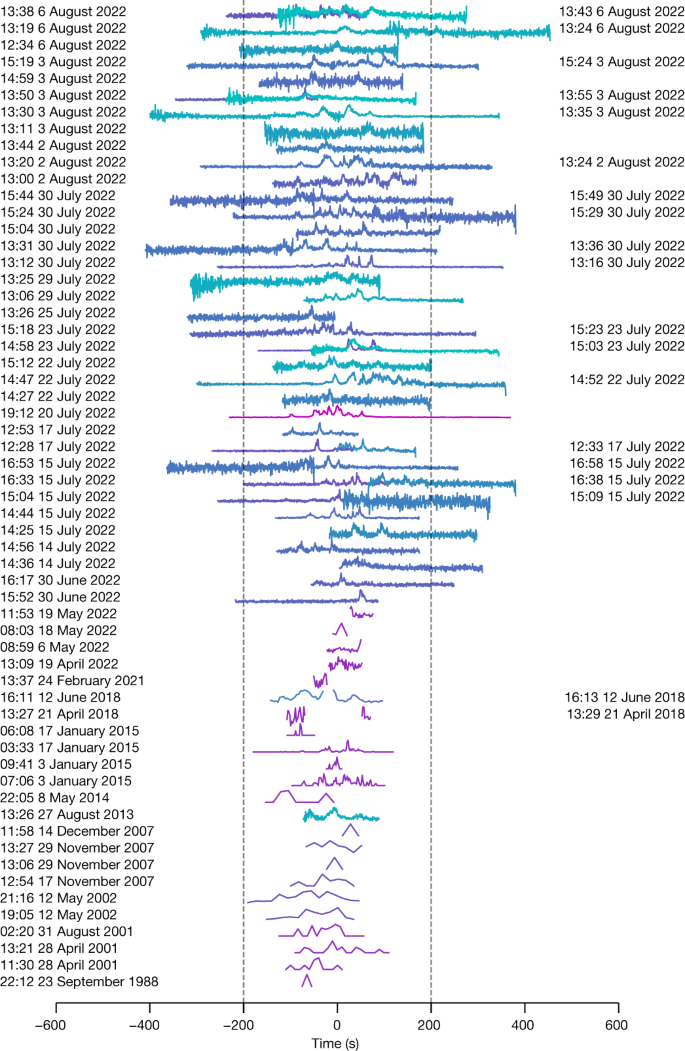
We measured the arrival times for all the detected pulses spanning nearly 34 years and, using standard pulse-timing methods, we derive a period P of 1,318.1957 ± 0.0002 s. Despite the wide variation of pulse-arrival times within the pulse phase, this large time lever arm also enables an estimation of the spin-down rate 𝑃., constraining it to ≲3.6 × 10−13 s s−1 (1-σ limit).
Figure 3 shows the radio flux density spectrum of a typical bright pulse; the radio luminosity of a pulsar-like source generating this pulse is 1028 erg s−1. This luminosity is larger than the available spin-down luminosity in the neutron star case, similar to GLEAM-X J162759.5–523504.3. However, this discrepancy has previously been seen in magnetar single radio pulses.
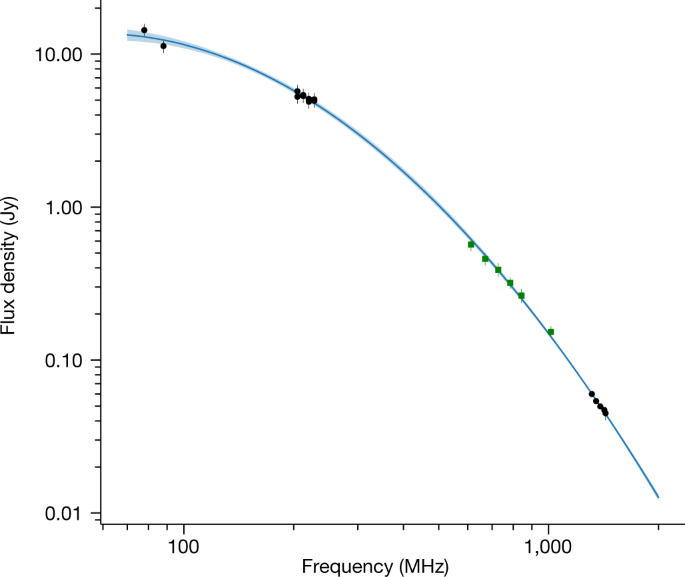
Coherent radio emission from rotating neutron stars has been explained by efficient pair production in the magnetosphere triggered by an accelerating region in the polar cap. Previous emission models have considered curvature radiation or inverse Compton scattering photons as the source of pairs; dipolar, multipolar and twisted magnetic fields; and vacuum-gap or space-charge-limited flows. Considering all these effects, there is a range of parameters (known as ‘death valley’) below which pair production is no longer an efficient mechanism and coherent radio emission is no longer expected. In Fig. 4, we plot the period derivative as a function of the spin period for different classes of isolated neutron stars. To encompass the largest parameter range, we have overplotted several ‘death valleys’ considering a few extreme cases. GPM J1839–10 falls at the very edge of the most generous death line. On the basis of our constraints on 𝑃., a classical coherent radio pulsar emission from a rotating neutron star is barely viable.
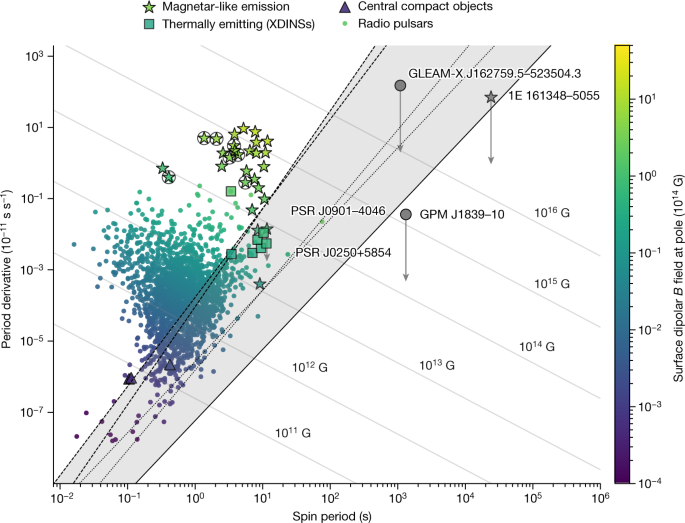
The long-lived activity of GPM J1839–10 is extremely puzzling. The radio activity of the P = 18.18-min transient GLEAM-X J162759.5–523504.3 was short-lived, persisting for only three months (with no detected nulling episodes) in 8 years of observations, with no further detections in archival data or recent monitoring. This led to the postulation that the radio emission could have been powered by a temporary rearrangement of its magnetic fields, preceded by a(n unobserved) high-energy outburst, similar to canonical magnetar radio emission. PSR J0901–4046, a postulated ultra-long-period neutron star pulsar with a rotational period of about 76 s, has been continuously active since its discovery, but its well-constrained 𝑃. places it above the death lines for several pulsar-emission-mechanism models(see also Fig. 4). By contrast, GPM J1839–10 has been active for at least three decades, although with a 50–70% nulling fraction. This new source has neither a short enough period to be explicable by canonical radio pulsar emission nor a short enough activity window for its radio emission to resemble a typical magnetar outburst.
An observation with XMM-Newton simultaneous to the ASKAP observation did not detect X-ray emission from the position of GPM J1839–10. Extended Data Fig. 1 shows the limits on the persistent luminosity in the soft X-rays assuming both thermal and non-thermal spectral shapes. For a reasonable range of parameter values, the derived values lie in the range (0.1–1.5) × 1032 erg s−1. Two very bright radio pulses were recorded by the ASKAP during the X-ray observation, with no X-ray counterparts (see Extended Data Fig. 2). We derived limits on the X-ray luminosity during the bright radio pulses on the order of <2 × 1033 erg s−1. These limits are orders of magnitudes below those measured for magnetar-like X-ray bursts. These results rule out a direct connection between these radio bursts and magnetar-like X-ray events, as in the case of SGR 1935+2154. GPM J1839–10 was also observed in the infrared Ks band with the Espectrógrafo Multiobjeto Infra-Rojo (EMIR) mounted on the 10-m Gran Telescopio Canarias (GTC). A faint source was marginally detected at Ks = 19.7 ± 0.2 (see Extended Data Fig. 3). However, the present data do not allow us to firmly conclude whether the source is single or blended, or if it is truly associated with the radio source.
There are several alternatives to the neutron star (radio pulsar or magnetar) interpretation. A rotating and highly magnetic isolated white dwarf, with its larger moment of inertia, could produce pulsar-like radio emission. However, it is perhaps surprising that no close-by highly magnetic white dwarfs have been observed to produce such emission. The only known white dwarf pulsar emitting in radio, AR Sco, has a P = 2-min spin period and a binary companion in a 3.5-h orbit. However, this radio emission, partly produced by the interaction with the companion star, is three orders of magnitude less luminous than the emission from GPM J1839–10. A highly magnetic rotating proto-white dwarf (or ‘subdwarf’) is a possibility and it could potentially be obscured by its red giant progenitor; both obscured and unobscured cases could be tested with further optical and infrared observations. Low-frequency radio emission has also been detected from star–exoplanet interactions and brown dwarf binaries, with modulations on both rotational and orbital periods. However, such emission is markedly circularly polarized and is around eight orders of magnitude less luminous than the emission observed from GPM J1839–10.
The detection of GPM J1839–10 and GLEAM-X J162759.5–523504.3 confirms that ultra-long-period radio sources are not extremely rare and there will be many opportunities in upcoming galactic-plane surveys to find further examples. Correlation of the observed population with predicted spatial distributions may yield further insights. Higher-frequency radio observations will be less affected by scattering, but if the radio flux density spectrum of these sources is identical, then detecting them beyond 1.4 GHz will be extremely challenging. Classical coherent radio emission mechanisms involving an accelerating potential gap and subsequent pair production do not seem viable to explain GPM J1839–10 (see N.R. et al., manuscript in preparation, for further discussion, including the magnetic white dwarf scenario). Intriguingly, the persistence of GPM J1839–10 over three decades indicates that there may be many more long-lived sources, some of which may await discovery in existing archives. Sources similarly active for decades, but with smaller pulse windows, would yield better constraints on 𝑃. and thus even stronger tests of models of coherent radio emission from astrophysical sources.